Swim-training affects zebrafish development: from molecules to function
In the last decades, it became clear that not only molecular signals but also mechanical forces are crucial regulators of developmental processes. The molecular mechanisms via which mechanical forces mediate their control of developmental processes have been extensively investigated via in vitro studies. However, the full range of molecular signals and mechanical forces that regulate development in vivo cannot be covered with only in vitro studies. In vivo studies have just started to reveal the molecular link between mechanical forces and development.
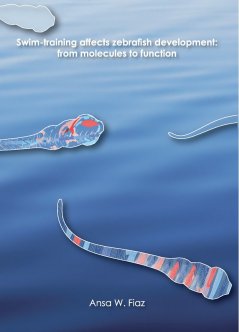
To help fill this gap, we subjected zebrafish larvae to swim-training, which presumably increases mechanical load, and investigated the effect thereof on a molecular, morphological and kinematic level during development. The zebrafish is an established model for vertebrate development and has a reproduction cycle of only three months, transparent embryos and starts to swim as early as 2 days post fertilization. A wide range of genetic analyses and tools to investigate developmental processes at various stages (embryo, larval, juvenile, adult) and levels (e.g. molecular, cellular and organ level) are available for the zebrafish.
To investigate the effect of the applied flow on the swimming kinematics of larval zebrafish, we performed a detailed analysis of the swimming kinematics of zebrafish larvae in varying flow conditions at 10 days post fertilization (dpf). We used a two camera set-up (obtaining recordings from the lateral and bottom side of the tube) and a new in-house developed 3D tracker program which allowed us to take pitch and roll angles into account and to accurately track the motion of the center of mass, the lateral bending of the body and motion of the tail fin. Furthermore, our study is the first which calculated the actual swimming speed of larval fish subjected to flow by determining the ambient flow velocity near the position of the fish via particle image velocimetry and computational fluid dynamics analysis of the flow in the tube. The analysis of the swimming kinematics showed that fish subjected to flow had a higher tail beat frequency and swam faster and longer. In addition, normalized maximum curvatures during an individual swimming burst (a forward movement consisting of several tail beats) and averaged over the first three half beats were higher in the caudal fin in fish subjected to flow. These data indicate that the applied flow increased the swimming intensity during a swimming burst and time spent on swimming.
Subsequently, we investigated the effect of swim-training on chondrogenesis and osteogenesis of the cranial, axial and appendicular skeleton during early larval development (5-14 dpf). Swim-training prioritized the development of cartilage and bone structures in the head and in the median fins. We furthermore demonstrated that swim-training can increase growth depending on the feeding regime, training regime and stocking density of fish in a tube during zebrafish larval development. Furthermore, swim-training increased burst frequency which indicates that swim-training not only increased swimming intensity but also swimming activity.
To investigate the effect of swim-training on the gene expression during zebrafish larval development, we performed transcriptome analysis of zebrafish larvae subjected to training and control fish at 10 dpf on a quantitative and spatial gene expression level. We chose this stage because the first ossified structures (hypurals and finrays) appear in the caudal fin around 10 dpf. This analysis demonstrated that swim-training led to molecular changes in the brain, muscle and gastrointestinal system during zebrafish larval development. In addition, we investigated if swim-training affected the expression of genes involved in muscle growth and structure with quantitative real-time PCR in trained and control fish at 5 and 14 dpf. The expression of slow fiber markers was increased after ten days of swim-training, indicating that muscle can already shift towards a slower aerobic phenotype during zebrafish larval development.
Furthermore, since swim-training prioritized the onset of skeletal elements in (among others) the caudal fin and fish subjected to flow had higher normalized maximum curvatures in the caudal fin, we explored the molecular link between an increased swimming activity and caudal fin development. Fish were subjected to swim-training from 5-10 dpf and we performed a whole genome microarray analysis on the caudal fins of fish at 10 dpf. From this analysis, we identified two muscle specific genes, aste1 (asteroid homolog 1) and zgc:65811, which showed an increased expression exclusively in the caudal fin. The gene aste1 contains an XPG coding domain and might be involved in DNA repair in muscle fibers. Since DNA repair plays an important role during cell division, this suggests that this gene might be implicated in cell division in muscle fibers. The gene zgc:65811 contains a domain which encodes for the CD-9 large extracellular loop region. The protein CD-9 belongs to the family of tetraspanin transmembrane proteins and is possibly involved in muscle fiber differentiation.
Our study demonstrated that swim-training can increase growth and lead to morphological and molecular changes during zebrafish larval development. We furthermore showed that the applied flow generated larger peak curvatures in the caudal fin and that swim-training led to specific morphological and molecular changes in the caudal fin. Our work suggests that the zebrafish is an interesting model species to elucidate the in vivo molecular link between functional performance and morphological development.