How do birds sing?
Sound analysis - mechanical modellling - muscular control.
Communication is a main key to survival. Many animals use sound to exchange information, which is important in species recognition, sexual selection and consequently in speciation. The animals best known for their vocal virtuosity are birds, which use acoustic signals extensively to communicate. Bird song supplies an important model in the study of learned vocal communication including human speech, animal behaviour and speciation. To produce sound, birds have evolved an organ unique in the animal kingdom; the syrinx, which is located at the bifurcation of the trachea into the bronchi. Although the syrinx was already identified as the sound source in the 18th century, the principle mechanisms that generate the sound are still under debate. Unravelling the physiology of sound production faces tremendous experimental challenges despite improved technologies due to its inaccessible location in the birds' body.
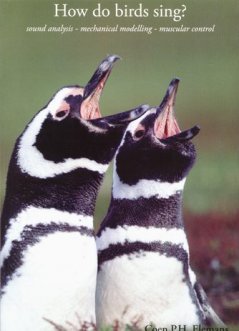
In this thesis, we focussed on the mechanisms underlying sound production and explored three approaches to improve understanding of both sound production and vocal control in birds. First, we explored what sound signals can teach us about the underlying mechanical events using a theoretical signal analysis approach.
Second, we present the first mechanical model of the syrinx that facilitates detailed study of membrane vibrations. As inception we used a relatively simple non-oscine syrinx of the family Columbidae. Our model consisted of a tube with a single membrane in its casing. The adduction of the membrane was controlled by the pressure in a simulated air sac relative to the driving pressure in the tube. We used the Laser Doppler Vibrometry (LDV) technique to measure membrane vibrations, and also measured air sac and bronchial pressures in our model. The functional window for sound production was determined by the position of the membrane. Without an initial complete closure of the tube by the membrane, no membrane oscillations were induced, and no sound was produced. The vibrations of the membrane were coupled to the resonance properties of the distal tube, but membrane material properties and tension also alterd the frequency of the sound.
Third, we studied the contractile properties of the syringeal muscles that control sound production in the ring dove (Streptopelia risoria). We showed that the syringeal muscles of ring doves are highly specialised superfast muscles. We simultaneously recorded sound and muscle activity with electromyography in vivo and showed that the syringeal muscles gate and frequency-modulate individual sound elements of the trill. Additionally, we measured the in vitro contractile properties of the syringeal muscles. These force measurements confirmed the superfast twitch characteristics of the syringeal muscles. The discovery of this special muscle type in the syrinx implied that superfast muscle can no longer be considered a rare adaptation and are probably the main muscle type controlling birdsong.
We also investigated the non-isometric properties of the syringeal muscles in ring doves using the workloop technique. The capabilities of the syringeal muscles strongly supported the notion that they act as antagonists for the positioning of the sound-producing structures, the lateral tympaniform membranes. The morphology of the syringeal muscles at ultra-structural level was typical for muscles that are superfast and require a high endurance. We observed large quantities of mitochondria, sarcoplasmatic reticulum and two T-tubuli per sarcomere in both muscles. Our results provided strong evidence that the syringeal muscles are the main frequency modulating structures of song in ring doves. Furthermore, our in vitro results on force modulation serve as essential input to state-of-the art mathematical models of sound production in birds.